Swarms of small satellites could communicate amongst themselves to collect data on important weather patterns at different times of the day or year, and from multiple angles. Such swarms, using machine learning algorithms, could revolutionize scientists’ understanding of weather and climate changes.
Engineer Sabrina Thompson is working on software to enable small spacecraft, or SmallSats, to communicate with each other, identify high-value observation targets, and coordinate attitude and timing to get different views of the same target.
“We already know that Saharan dust blowing over to the Amazon rainforests affects cloud formation over the Atlantic Ocean during certain times of the year,” said Thompson, who works at NASA’s Goddard Space Flight Centre. “How do you capture that cloud formation? How do you tell a swarm of satellites what region and time of day is the best to observe that phenomenon?”
Under Thompson’s plan, scientists would establish a set of requirements for observations and define high-value targets. Then the software would take over, enabling a spacecraft swarm to figure out how to move relative to one another to best observe these targets. Strategies might also change based on time of day, season, or the region being observed. The spacecraft also would use onboard machine learning to improve viewing strategies over time.
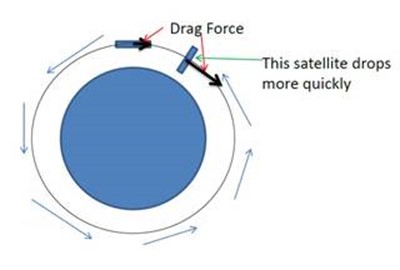
Setting the following spacecraft to maximize drag and the leader to minimize drag will cause the follower to drop in altitude and catch up to the leader.
Credits: NASA/Sabrina Thompson
“There are several types of swarm configuration being considered,” Thompson says. “One might be a swarm where satellites will be in different orbits, which will allow them to view a cloud or other phenomenon at different angles. Another swarm could view the same phenomena with similar view, but at different times of the day. A third type of swarm might combine both, with some satellites in the same orbit, following one another with some time offset, and other satellites which may be in orbits with different altitudes and/or inclinations.”
While a swarm would stay within the same orbit, individual spacecraft could even use something called differential drag control — manipulating the forces caused by Earth’s atmosphere dragging against the orbiting craft — to control the time separation between each spacecraft relative to others in the swarm, she says. “The length of time it takes to perform a differential drag maneuver depends on the spacecraft mass and area, as well as the orbital altitude. For instance, it can take as long as one year or as short as a couple of days, even hours.”
“With multiple spacecraft in one formation to view the same target, you can see a cloud, for instance, not just from the top, but from the sides as well.” In a different formation, you can see that cloud at different stages of its life-cycle from multiple SmallSats passing at different times.
Working with University of Maryland – Baltimore County (UMBC) professor Jose Vanderlei Martins, Thompson helped develop the Hyper-Angular Rainbow Polarimeter (HARP) CubeSat that launched from the International Space Station (ISS) just over a year ago. An updated version of its instrumentation, called HARP2, will fly on the Plankton, Aerosol, Cloud, ocean Ecosystem (PACE) mission planned for launch in 2023.
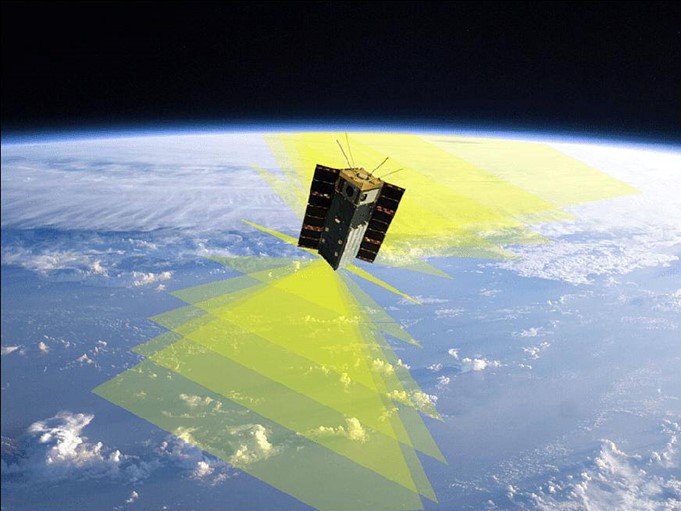
A SmallSat like this one, working with a swarm of similar spacecraft with more narrow-angle, high-resolution polarimeters, could potentially revolutionize understanding of weather formation and processes.
Credits: NASA/SDL/Jose Vanderlei Martins
A swarm of SmallSats like HARP, sharing information and coordinating coverage, could advance weather forecasting, disaster reporting, and climate modeling in the long term, Vanderlei Martins says. To get there, scientists need the combination of wide and narrow fields of view and high-resolution imagery to better understand the dynamics of weather system development.
“Ideally, I like to have a satellite with a wide field of view observing larger phenomenon,” he adds. “However, a small satellite covering a large area cannot make high spatial resolution observations. Nevertheless, you can use it as a surveyor type of satellite to identify the area of interest. Then you have others with a narrower field of view, getting higher resolution, getting much more detail.”
Enabling the swarm to make decisions and share information is crucial. Vanderlei Martins says. “These sorts of decisions need to be made in minutes. You don’t have time for ground control to be involved.”
Thompson notes that reducing reliance on ground control and communications networks also frees up resources for SmallSat missions with limited budgets.
As an aerospace engineer working towards an atmospheric physics degree at the University of Maryland, Baltimore County, Thompson went back to school to learn more about the Earth science requirements that drive her work as an innovator. “I also really wanted to understand climate change.”
How aerosol particles and clouds interact is crucial to understanding climate change. Polarimeters can provide a wealth of data about particles suspended in the atmosphere — from smoke, ash, and dust to water droplets and ice, each species of particle polarizes light reflected from it in detectable ways.
“At a basic level, my research involves evaluating the geometry between instruments on the satellite and the sun,” Thompson says. “These instruments are passive. They require a certain geometry relative to the ground target and Sun to retrieve the science data we want.”
Her algorithms will determine the most suitable combinations of orbit and instrument field of views to give the largest probability of observing a cloud with the appropriate geometry to retrieve science data. Then it would plan and execute maneuvering schemes for each spacecraft to achieve those geometries relative to the other satellites in the swarm.
This work to understand the structure and development of clouds ties in with the Atmosphere Observing System, or AOS, (formerly the Aerosols and Clouds, Convection and Precipitation study identified as a priority in the 2017 Earth Decadal Survey. Vanderlei Martins and Thompson believe their swarm technology complements AOS’s science objectives and could enhance upcoming NASA Earth science missions.
Featured picture: Two satellites on similar orbits collect valuable perspectives on the same part of the atmosphere.
Credit: NASA/Sabrina Thompson